What our lab researches
Our lab determines how molecules and proteins shape the functions of immune and heart cells by blending computational science and traditional wet-lab experiments. Our projects encompass an exciting journey investigating molecular mechanisms underlying chronic pain, Alzheimer's, anti-tumor therapies, and cardiac disease. We specialize in probes of calcium-dependent proteins like troponin C and calmodulin using advanced techniques such as molecular simulations and live-cell microscopy. Our diverse team is highly interdisciplinary and collaborates globally, thereby creating an environment for groundbreaking discoveries.What is computational science?
Computational science is a frontier where cutting-edge computing techniques, powerful algorithms, and simulations combine to unravel the mysteries of how living cells work. These approaches provide a virtual environement to explore the inner workings of cellular systems that would otherwise be challenging, exorbitantly expensive, or simply impossible to dissect directly. Computational scientists use multidisciplinary knowledge, high-performance computing, and mathematical models to add new dimensions to what we study via traditional bench-top experiments.Our Current Projects
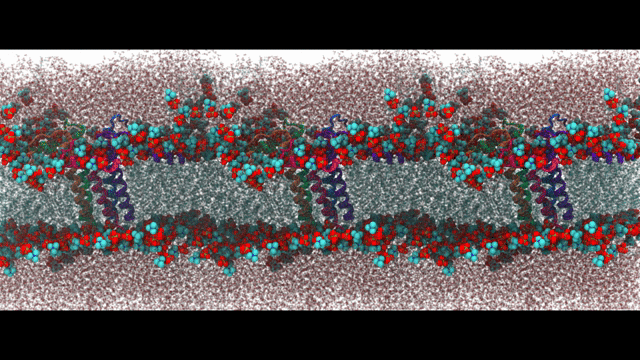
Engineering proteins to control calcium signal transduction
Calcium is a molecule that proteins use to communicate and perform work. When calcium regulation is disrupted in cells it can lead to various diseases like Alzheimer's and chronic pain. To address this issue, my lab has been researching how to engineer calcium-binding proteins to counteract disrupted calcium signaling. However, engineering these proteins has been a challenging and largely ineffective process, despite the availability of abundant structural data of these proteins. Our hypothesis is that by controlling the changes in structure or solvent-exposed surface that occur during calcium binding, we can significantly modify calcium affinity, even if not the binding kinetics. To tackle this challenge, we are using an innovative approach that combines statistical physics models of ion binding and protein interactions with 3-D protein structures, binding poses, and interaction energies obtained from molecular simulations at the atomic level. We are complementing these studies with traditional cell biology to investigate the function of the engineered proteins. Our related publications can be found here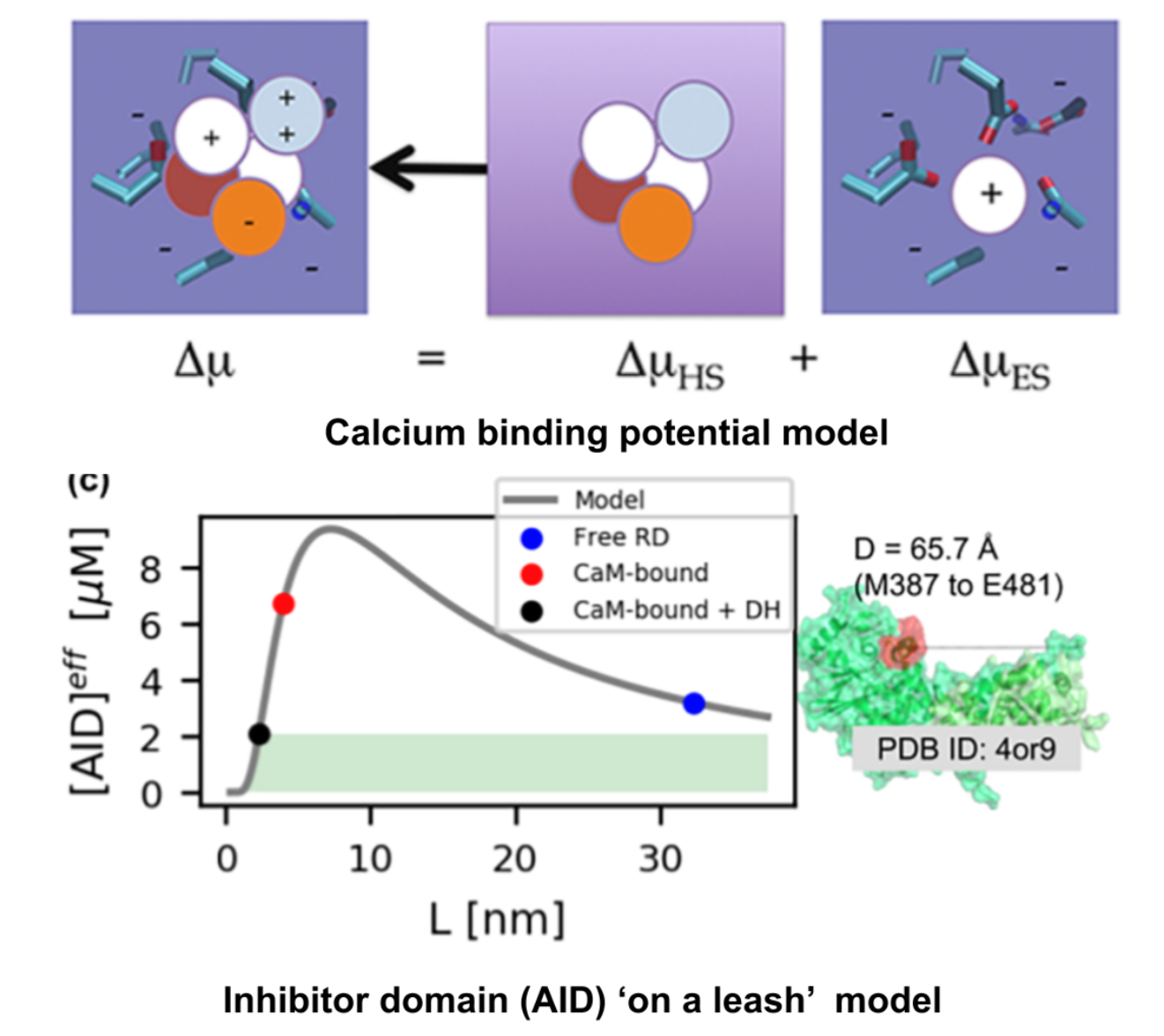
Immune responses in microglia and macrophages
Pro-inflammatory responses in macrophages rely on several calcium-dependent signaling pathways working together. To understand these mechanisms better, we are improving our system biology modeling to investigate similar calcium signaling pathways in inflammation-related macrophages. However, our knowledge of macrophages' calcium signaling is often limited to non-physiological manipulations such as gene knock-out or knock-in, or treatments with potent inhibitors, which do not accurately reflect in vivo calcium signaling mechanisms underlying macrophage inflammatory responses. Although similar challenges have been overcome in computational systems modeling for cardiomyocytes, models for macrophages have not kept pace. Given the numerous experimental studies linking calcium to macrophage inflammatory responses, we hypothesized that triggering a small number of calcium-dependent signaling pathways through ATP-dependent activation of P2X channels, a type of transmembrane receptor, could lead to cytokine synthesis. To test this hypothesis, we created one of the first computational models of microglia, which are the resident macrophages in the central nervous system. We have validate this model in a mouse microglia cell line and are pursuing additional unresolved questions of calcium signaling in these cells. See related publications here.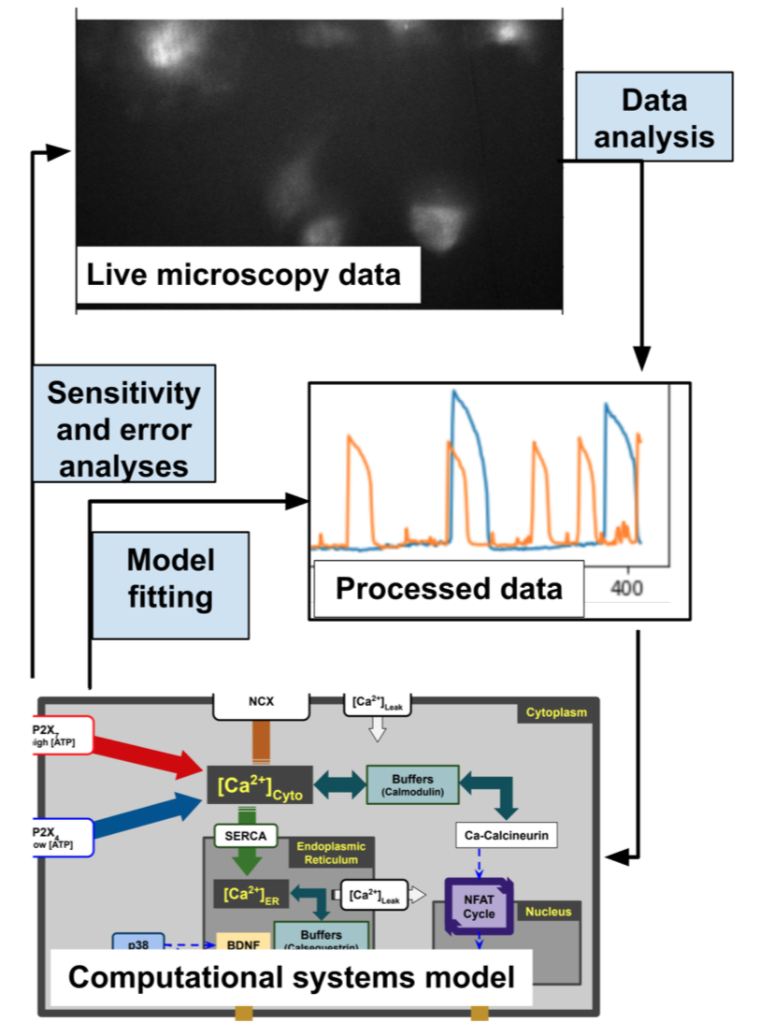
Understanding extracellular control of intracellular signaling pathways
Calcium binding proteins respond to changes in calcium levels in cells to facilitate calcium signaling. This signaling is often triggered by extracellular molecules such as ATP binding to transmembrane receptors. However, triggering of receptors in vivo is more complicated due to various factors such as intercellular junction composition and nucleotide metabolism. To investigate this, we developed a computational model of diffusion to predict how ATP signals propagate to junctional receptors in vivo and trigger calcium fluctuations. However, most existing models either lack the spatial accuracy or atomistic-scale interactions to capture the entire signaling process. To address this, our lab has developed a multi-scale approach that models signaling as reaction-diffusion processes occurring over large micron-sized regions, using effective parameters estimated from much smaller sub-regions. We are testing these concepts in the wetlab by observing how cells migrate in response to chemoattractants. Related publications can be found here.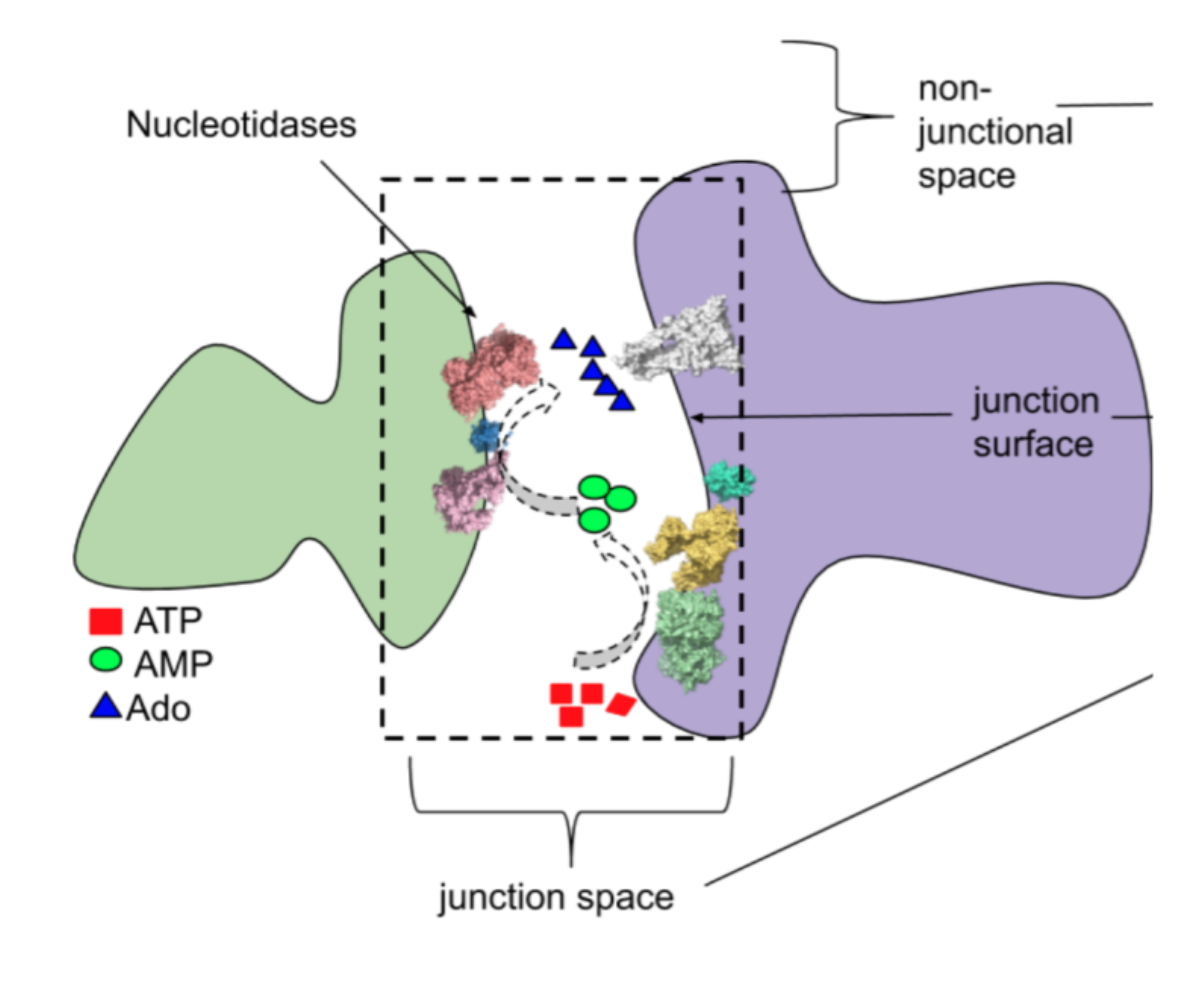